Proteins and Polypeptides
Proteins are organic compounds that contain four elements: nitrogen, carbon, hydrogen, and oxygen. To comprehend the full scope of proteins, it is crucial to understand various properties, including the basic biological molecule, peptides, polypeptide chains, amino acids, protein structures, and the processes of protein denaturation.
Polypeptides and Proteins: What's the difference
According to IUPAC, polypeptides with a molecular mass of 10,000 Da or more are classified as proteins. At times, the term 'proteins' refers to molecules with 50-100 combined amino acids. Each protein contains one or more polypeptide chain. The chemical properties and order of the amino acids determines the structure and function of the polypeptide. Each of these polypeptide chains is made up of amino acids, which are joined together in a detailed, deliberate and specific order.
What do proteins do for the body?
Proteins play vital roles in human biochemistry, primarily serving as the body's building blocks. They act as precursors to various biologically relevant molecules, and imbalances—either excess or deficiency of proteins—can lead to diseases, resulting in nervous system defects, metabolic issues, organ failure, and even death.
Proteins exhibit a diverse range of functions in the body, which can be categorized alphabetically:
Antibodies: These proteins bind to specific foreign particles, such as viruses and bacteria, contributing to the body's defense. Example: Immunoglobulin G (IgG).
Enzymes: Responsible for executing the multitude of chemical reactions within cells, enzymes also assist in the formation of new molecules by interpreting genetic information from DNA. Example: Phenylalanine hydroxylase.
Messenger Proteins: Some types of hormones fall into this category, transmitting signals to coordinate biological processes among different cells, tissues, and organs. Example: Growth hormone.
Structural Components: Providing structure and support for cells, these proteins also enable bodily movement on a larger scale. Example: Actin.
Transport/Storage: These proteins bind and carry atoms and small molecules within cells and throughout the body. Example: Ferritin.
As proteins are essential for cell and tissue growth, ensuring an adequate protein intake is particularly crucial during periods of rapid growth or increased demand, such as childhood, adolescence, pregnancy, and breastfeeding
Protein Sources
Protein-rich foods come from both animal and plant sources. Here are examples of high-protein foods. The values are given per 100 grams of the food:
Animal-Based Proteins:
Chicken Breast (cooked, skinless): ~31 grams
Salmon (cooked): ~25 grams
Ground Beef (cooked, 90% lean): ~26 grams
Eggs: ~13 grams
Greek Yogurt: ~10 grams
Milk: ~3.4 grams
Cheese (cheddar): ~25 grams
Plant-Based Proteins:
Lentils (cooked): ~9 grams
Chickpeas (cooked): ~8 grams
Black Beans (cooked): ~8 grams
Quinoa (cooked): ~4 grams
Tofu: ~8 grams
Almonds: ~21 grams
Peanut Butter: ~25 grams
While both animal and plant-based proteins provide essential amino acids, there are some distinctions:
1. Amino Acid Profile: Animal proteins generally contain all essential amino acids in the right proportions, making them "complete" proteins. Most plant proteins lack one or more essential amino acids, making them "incomplete." However, combining different plant protein sources throughout the day can provide a complete amino acid profile.
2. Digestibility: Animal proteins are often more easily digestible than some plant proteins. However, cooking and preparation methods can enhance the digestibility of plant proteins.
3. Nutrient Content: Animal products usually contain additional nutrients like vitamin B12, heme iron, and omega-3 fatty acids, which may be less abundant in plant sources.
Recommended Daily Protein Intake. The amount of protein a person needs can vary based on factors such as age, sex, physical activity, and overall health. However, a general guideline is:
Adults: The Recommended Dietary Allowance (RDA) for protein is 0.8 grams per kilogram of body weight. For example, a sedentary adult weighing 70 kilograms would need around 56 grams of protein per day. Athletes and those engaging in heavy physical activity may require more.
It's important to note that individual protein needs can vary, and consulting with a healthcare professional or a registered dietitian can provide personalized recommendations based on specific health goals and conditions.
Protein Basics
Proteins are among the most abundant organic molecules in living systems and are vastly diverse in structure and function as compared to other classes of macromolecules. Even one cell contains thousands of proteins, which function in a variety of ways. Proteins are composed of 20 different amino acids, for which genomes dictate the specific amino acids and their sequences.
As a result, variances in protein activities and functions occur due to the complex structural and functional properties of long-evolved proteins. Such properties include:
1. The process of rapid protein folding.
2. Binding sites specific to small groups of molecules.
3. Balanced flexibility in structures and rigidity levels in order to maintain protein functions.
4. Congruent protein surface structures suitable for a protein's environment.
5. Protein vulnerabilities to degradation cause damage, thus rendering protein useless.
Several structural materials are contained within proteins that exist in all living organisms. The many diverse functions relative to these structures are:
• Catalysis
– Increases rates of chemical reactions within proteins (e.g. enzymes).
• Structural
– Strengthens bones, tendons and skin (e.g. collagen).
• Movement
– Muscle contractions permit movement (e.g. myosin).
• Metabolic regulation
– Joining or taking biomolecules that are needed by the cell.
• Transport
– Moves oxygen throughout the body (e.g. hemoglobin).
• Defense
– Protein antibodies serve as protection against foreign pathogens (e.g. immunoglobulin).
Proteins form in different shapes and structures. Some proteins are globular (spherical), compact and water-soluble, while other proteins are fibrous and elongated, physically tough, and water-insoluble. Chemical bonds are responsible for the shapes that proteins maintain.
The 4 Protein Structures
Four different structures of protein serve to influence specific protein activities. Different sequences of amino acids form different shapes and thus, different proteins. All protein activity is based on these four structures:
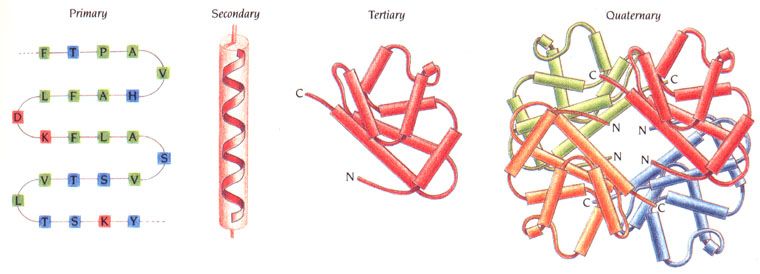
Primary Structure: The primary structure of a protein is its amino acid sequence. The base (repeat) sequence of the gene codes are comprised of three amino acids (glycine; proline; «x» - any other amino acid). This sequence of amino acids bonded together creates a polypeptide (poly = many) bond, or chain. The primary structure of a protein is linear.
Secondary Structure: The secondary structure takes the chains (primary) and folds, or coils them. These parts attract to one another to form structures that have α(alpha)-helices and β(beta)-pleated sheets. These form as a result of hydrogen bonds between the peptide groups of the main (primary) chain. These secondary-structure proteins contain regions that are cylindrical (α-helices, spiral shape) and/or regions that are planar (β-pleated sheets, ribbon with peaks and valleys). The secondary structure of a structure is three dimensional.
Tertiary Structure: The tertiary structure of a protein is its three-dimensional conformation that is created when the protein folds. Hydrogen bonds stabilize the folding occurrences. Other intramolecular bonds that stabilize the folding processes include hydrophobic interactions; ionic bonds; and disulphide bridges. These bonds are formed between the R groups of amino acids. They contain the nonpolar parts of proteins which result in attractions and repulsions and become coiled up in one area, creating a very complex structure. The tertiary structure is the overall shape of the protein for which most are globular in shape, or fibrous – long and thin.
Quaternary Structure: A quaternary structure is formed when two or more tertiary polypeptide chains form a single or full protein. Certain proteins may have a non-polypeptide structure, thus belonging to a prosthetic group, while other proteins are conjugated. Here unique patterns are formed via hydrogen bonding.
Chemical Bonding in Proteins
The amino acids of a polypeptide are linked together in chains by their neighboring covalent bonds (peptide bonds). In turn, each bond forms in a dehydration synthesis (condensation) reaction. Throughout the protein-synthesis process, the carboxyl group of the amino acid reacts with the amino group of an incoming amino acid, releasing a molecule of water. This process occurs at the end of the growing polypeptide chain, thus resulting in a bond between amino acids, known as a peptide (or polypeptide) chain.
In addition to the polypeptide bonds, sulfide bridge formations and Schiff base formations are two other chemical reactions that can take place during cell activities that are «directed» by amino acids, which comprise the basis of all proteins.
The amino acids' interactions cause a protein to fold into its mature shape (tertiary). Such folding happens because of the rotation of bonds within the amino acids, as well as bonds joining various amino acids.
Peptide bonds form between the carbonyl carbon and the nitrogen, which is another peptide bond. Nitrogen bonds to carbonyl carbon, then to the peptide linkage, back to the nitrogen, and so on. From the chains, the backbones are interacting. Furthermore, nitrogen is electro-negative such that it consumes electrons from the nitrogen. Here the hydrogen has a partially positive charge.
Conversely, oxygen is electro-negative. Oxygen takes electrons from the carbon which gives oxygen a partially negative charge. Simply put, the hydrogen and the oxygen are attracted to each other, creating a hydrogen bond. From there, these two connected chains form a β-pleated sheet. The backbones then interact with each other and move in the same direction, which is known as a parallel β-pleated sheet.
The β-pleated sheet takes on a certain pattern, moving in the order of nitrogen, α carbon, and then carbonyl carbon. This is on the left side of the protein. On the right side, the pattern moves in the order of carbonyl carbon, α carbon, and then nitrogen. Note that these processes are traveling in opposite directions with the hydrogen bonds between these partially positive ends of the nitrogen-hydrogen bonds remaining at the hydrogen end. The hydrogen bonds and the backbones are still parallel, but again, they are moving in different directions. This is called an anti-parallel β-pleated sheet, which is another form of a secondary structure.
The backbone of the protein can also become a helical structure (also a secondary structure) where the hydrogen bonds form between the different layers of the helix. Remember that oxygen has a partially negative charge, and that hydrogen has a partially positive charge. At any rate, the resulting hydrogen bond gives the protein its helical structure. So these interactions highlight the in-depth and interrelational processes that create the structure, dynamics, and the functions of proteins.
All proteins have the above-mentioned processes in common, but not all proteins possess the more complicated and interactive tertiary and quaternary structures. Several molecular interactions and thermodynamic changes can transpire within these highly complex molecular structures of protein.
Protein Denaturation
Protein denaturation occurs when the three-dimensional structures (in secondary, tertiary, or sometimes quaternary structures) of proteins is altered. Denaturation makes protein non-functional, or at least unable to perform its usual functions. However, the peptide chains are left intact following denaturation, as are the chemical properties of protein. Most usually, the denaturation process is irreversible.
Factors such as heat, cold, or unfavorable pH can cause protein denaturation resulting in damage between the molecular structures of the peptide bonds, thus breaking the otherwise covalent bonds. «Healthy» proteins fold over one another, but denaturation causes them to unravel.
To understand how factors such as high temperature and high or low pH cause denaturation, it is important to remember the fact that hydrogen bonds are crucial in the secondary, tertiary, and sometimes the quaternary structures of protein. A hydrogen bond is a type of peptide bond. Carbon, oxygen, and nitrogen are equally essential in the aforementioned protein structures.
Too much heat, for example, will result in the twisting, bending and rotating of functional molecular groups, and since hydrogen is known to be fragile, it is more vulnerable to ruptures. Temperature increases disrupt the existing hydrogen bonds as well as other non-polar hydrophobic interactions. As the temperature increases, so does the kinetic energy. This causes the molecular components of the protein to vibrate, which leads to the broken bonds. This, in turn, creates a pattern of ruptures or breaks of any nearby hydrogen bonds.
References
1. Hegyi H, Bork P. On the classification and evolution of protein modules. J Protein Chem. 1997 Jul;16(5):545-51. [PubMed].
2. A. D. Mclachlan. Protein Structure and Function. Annu. Rev. Phys. Chem. 1972.23:165-192.
3. Jay R. Hoffman, Michael J. Falvo. Protein - Which is Best? J Sports Sci Med. 2004 Sep; 3(3): 118-130. [PubMed].
4. Kent SB. Total chemical synthesis of proteins. Chem Soc Rev. 2009 Feb;38(2):338-51. [PubMed].